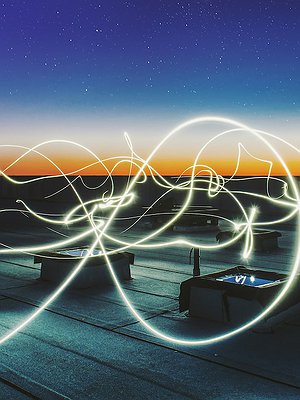
Author: КИСЛОВ КОНСТАНТИН АЛЕКСАНДРОВИЧ / KISLOV KONSTANTIN ALEXANDROVICH
Introduction
From the very beginning of the discovery of electricity, there was a problem of its transmission to the end user. The development of industrial production has led to a sharp increase in demand for electricity. Wires and poles of electric transmission lines have become an integral element of landscapes. The development of modern means of communication and mobile computing devices requires frequent recharging of their batteries. And the presence of each model of its own charger greatly burdens the charging process and eliminates the possibility of simultaneous connection of several electrical appliances. The relatively new wireless charging technology is just one of the many areas of wireless power transmission technology. Wireless transmission of electricity over a distance has been known since Michael Faraday discovered the phenomenon of electromagnetic induction in 1831. Experimenting with magnets, Faraday set himself a specific goal: to get electricity using magnetism. The scientist perceived magnets as a source of electricity - and his experiments were crowned with success. Faraday proved that when the magnetic flux passing through a closed circuit changes, an electric current arises in the latter. Nikola Tesla first proposed and demonstrated wireless power transmission as an alternative to transmission and distribution of electric lines in 1899 (Appendix Sheet I, Fig. 1). The most promising, in the direction of wireless transmission of electricity, is the induction method. Professor John Pendry of Imperial College London believes that such devices could well have been made 10 or 20 years ago. At the same time, he believes that the decisive role was played by the recent appearance of a huge number of portable devices that need constant energy supply: "The charger wire has become the last link in the wireless circuit to get rid of." At the moment, there are several standards that are already in effect and allow manufacturers to produce ready-made solutions. For example, the global international Qi standard developed by the Wireless Electromagnetic Energy Consortium (WPC) is aimed primarily at the domestic use of wireless chargers. Wireless charging systems can also be used to charge electric vehicle batteries. In this case, it is necessary to ensure an effective power transmission of more than 3 kW over a distance of 10-20 cm. To perform induction charging, it is enough to install the car above the transmitter, and the charging process will start automatically. It is expected that the use of wireless charging will contribute to the growing popularity of such vehicles. Wireless power transmission systems can also be used as an energy source for LED lighting in the room. In this case, LED lighting designers have ample opportunities to implement new solutions for hanging lamps, which, as it may seem, "float" in the air. In this case, for single lamps, it is enough to transmit a power of about 10 watts. One of the most important advantages of wireless technology is its use in explosive environments, since due to the lack of power connectors, the possibility of an explosion from a spark that may occur when the charger is connected or disconnected is excluded. Thus, the spheres of use of wireless energy transmission technologies are quite diverse and only one problem hinders the introduction of these technologies, small distances of energy transmission, and insignificant capacities. Solving this problem will allow us to move to a new level of designing electrical products and, in general, will change many production technologies.
Purpose and objectives of the work
In modern times, the direction of designing low-power wireless chargers for charging mobile equipment is developing. The basic principle of inductive charging is very simple and is based on electromagnetic induction. Unlike classic chargers, their wireless counterparts use an additional conversion unit instead of a copper conductor, similar to a conventional transformer, which leads to a certain decrease in the efficiency of this unit. According to different manufacturers, the efficiency ranges from 50% to 90%. Wireless Power Consortium calls an intermediate figure – 70%. The inductive charger is designed for the possibility of simultaneous "connection" of several devices. Unlike traditional chargers, accordingly, additional sockets will not be needed for this. Well, from the point of view of aesthetics, the absence of extra wires has always been a big plus. However, there are also significant problems in these studies, such as the consumption of induction energy of considerable power. Two years of development and research into the possibilities of wireless energy transmission have shown that it is quite simple to carry out the transmission of energy wirelessly over short distances. Several devices have been manufactured that make it possible to successfully wirelessly charge batteries at short distances between the transmitting and receiving coils. The efficiency of energy transfer in our devices did not exceed 50%. Having studied a considerable amount of literature and Internet sources, we came to the conclusion that there are real possibilities of increasing the distance of energy transmission by improving transceiver antennas, increasing the power and efficiency of transceiver devices, when they are coordinated, and tuned into resonance.
The purpose of the new work is: to study the possibilities of increasing the distance of wireless transmission of electricity due to the resonant matching of the receiving and transmitting coils, and while maintaining effective power indicators.
The goal was to solve the following tasks:
Acquaintance with literary and Internet sources on the issues of induction transmission of electricity.
Analysis of the characteristics of last year's developments of wireless charging stations.
Study of the principles of operation of high-frequency induction inverters of high power.
Manufacture and verification of characteristics of inverter devices for wireless power supply of energy consumers.
Study of the principles of calculation and coordination of the receiving and transmitting coils.
Carrying out experimental work to increase the distance of energy transmission by induction, using an inverter and resonant coils.
Wireless power transmission technology
The possible options for wireless power transmission technology used to power electrical appliances and charge batteries are quite diverse (Appendix Sheet I, Fig. 2). The first group of methods (far zone) refers to electromagnetic radiation. Electromagnetic waves surround us everywhere. They are emitted by a variety of radio frequency transmitters, TV towers, cellular communication antennas, industrial equipment. But the possibilities of using this energy are limited by its low power. For humanity, this is salvation, because powerful electromagnetic radiation is harmful to all living things. Thus, the most acceptable option for wireless power transmission is the second group of methods based on the phenomenon of electromagnetic induction. As is known, the electromagnetic field propagation area is divided into two main zones depending on the distance from the radiation source. The near zone (induction zone) is limited by a distance equal to λ/2π, where λ is the wavelength. The technique of wireless transmission by electromagnetic induction uses a near electromagnetic field at distances of about one-sixth of the wavelength. The energy of the near field itself is not radiating, but some radiation losses still occur. In addition, as a rule, resistive losses also occur. The induction zone gradually passes into the radiation zone (wave). At the borders of the near and far zones, a transitional intermediate zone is distinguished (Appendix sheet I, Fig. 3). If we consider the frequency characteristics of radiation, it is possible to determine the values of the near zone, according to these frequencies (Appendix Sheet II, Table 1). The dependence of the distance of the near zone (induction zone) on the frequency of electromagnetic radiation clearly shows a decrease in the distance of the near zone with an increase in the frequency of radiation. In wireless charging systems (as a simple case of wireless power transmission), the phenomenon of electromagnetic induction is used to transfer energy from the source (transmitter) to the receiver. In 1831 Michael Faraday discovered that an electric current occurs in a closed conducting circuit when the magnetic field changes. This current is called an induction current. You can change the magnetic field using a magnet or a coil. Thus, electromagnetic induction is a physical phenomenon of the occurrence of an electric current in a closed conducting circuit with changes in the magnetic field penetrating the circuit. The appearance of an electric current in a closed circuit with changes in the magnetic field penetrating the circuit indicates the action of external forces in the circuit or the occurrence of EMF induction. A quantitative description of the phenomenon of electromagnetic induction is given on the basis of establishing a connection between the EMF of induction and the magnetic flux F. In the SI system, the unit of magnetic flux is chosen such that the coefficient of proportionality between the EMF of induction and the change in magnetic flux is equal to one. Consequently, the law of electromagnetic induction is formulated as follows - the EMF of electromagnetic induction arising in a closed circuit is equal to and opposite in sign to the rate of change of the magnetic flux through the surface bounded by this circuit:
E_i=-(dP_m) / dt
Where: E_i is the electromotive force acting along an arbitrarily selected contour;
〖dF〗_m – small value of the magnetic flux;
dt is a short period of time.
The system consists of a primary coil L1 (source) and a secondary coil L2 (receiver). The coils form an inductive coupling system (a heartless transformer). Alternating current flowing in the winding of the primary coil creates a magnetic field that induces a voltage in the receiving coil, which can be used both to charge the battery and to power the device (Appendix Sheet II, Fig. 4). However, as the secondary coil is removed from the primary, most of the magnetic field dissipates and does not reach secondary coil. This is the main problem facing developers of wireless devices. Even at relatively small distances, the inductive coupling decreases so much that it becomes ineffective. The mutual inductance of the coils (M) is determined from the ratio:
where k is the coupling coefficient between the coils, depending on numerous factors (the distance between the coils (z), the ratio of the diameters of the coils, the displacement between their centers, the shape of the coils). Based on the graphs given in the appendix (sheet III, Fig. 5) it is easy to determine the coefficient (k) for coils with a diameter of 30 mm, spaced at a distance of 0.2 to 10 mm. Resonant circuits with inductive coupling used in wireless charging systems have been successfully used in a variety of radio engineering devices for decades. Currently, two industrial technologies have been developed for wireless energy transfer (charging) using the phenomenon of electromagnetic induction. One of them, which uses strongly coupled coils, is called MI (magnetic inductive), the other with weakly coupled ones is MR (magnetic resonance). These two technologies are similar, but there are differences. In each of them, an electromagnetic field is used for wireless transmission of energy, and resonant circuits are used. The magnetic flux generated by the electromagnetic field source and penetrating the secondary coil depends on the configuration of the field. The efficiency of the energy transmission system depends on the mutual orientation of the transmitting and receiving coils, as well as on the distance between them. The greater the distance between the coils, the less efficient the system is. In MR technology, several receiving coils are used to compensate for the displacement of the transmitting coil and the distance to it (Appendix Sheet III, Fig. 6). But such a solution significantly complicates the configuration of the resonant circuit and increases the dimensions of the device.
Circuit design of practical manufacturing of wireless transmitting devices and analysis of their effectiveness (based on last year's experiments)
Electric current is the ordered movement of free electrons from a negatively charged body (lack of positively charged electrons) to a positively charged one (excess of positively charged electrons). An electromagnetic field is formed around the conductor in which the electromotive force originated. The electromagnetic field is able to make free electrons move. Therefore, by moving a conductor in an electromagnetic field created by an external source (metal magnet, electromagnet, other conductors and electrical appliances containing them), it is possible to obtain a current flow. Strictly speaking, electricity does not come to our homes through wires, it is transmitted through an electromagnetic field around the conductor. In this case, part of the energy heats up the conductor itself, in which the electrons move, and part is scattered into space. The creation of the movement of electrons inside a conductor by crossing this conductor with magnetic field lines, as mentioned above, is called electromagnetic induction - the conversion of mechanical energy into electrical energy. An induction generator, an induction cooker, and an induction charger work exactly on this principle. A closed coil is installed in the energy source from a conductor through which current flows. The coil creates a vortex electromagnetic field, as the electrons inside the coil begin to move. A device that supports the wireless charging method has a similar coil that captures the vortex field of the source coil. Well, then everything is simple - the electromagnetic field creates an induction current - the movement of electrons - on the receiver coil in the device. It is important to note that the vortex electromagnetic field on the source coil is variable. That is, it oscillates, which causes the electrons in the receiver coil to move. To power the device, fluctuations must be smoothed by converting alternating current to direct current. To do this, the current from the coil passes through a special device - an electric current rectifier - in order to charge the battery in the form of a constant voltage current. The task of a high-frequency generator is to create an electromagnetic field in an inductor (transmitter coil). The basis of any induction wireless device is a high-frequency generator, transmitting and receiving circuit. Their production can be organized in various ways and with different element base. In general, the energy transmitter is a master generator and a sufficiently powerful key node that generates pulses to the transmitting frame. The simplest and cheapest solution is a circuit of a conventional multivibrator on two field-effect transistors (keys) (Appendix Sheet IV, Fig. 7). The transmitting frame is included in the drains of transistors with a parallel frequency-setting capacitor. The key transistors are field–effect, their expediency of use is determined by a significantly higher frequency and speed, as well as sufficient power. Such a circuit design has the advantage of automatically tuning the resonance in the transmission circuit L3-C1 and allows you to get significant power in the transmission circuit with low-voltage power supply. The receiving frame is tuned to parallel resonance by the capacitor C2. The load in our experiments was a resistive load (a light bulb and LEDs). The final experimental version of the circuit made according to the structure of the multivibrator is given in the appendix (Sheet IV, Fig. 8). High-power field-effect transistors designed for a pulse voltage of up to 500 volts were used in the manufacture. The need for this is caused by the fact that with subsequent measurements of the voltage at the output of the keys without load, the voltage reaches 200 volts. A significant frequency of the multivibrator (50-60 MHz) assumes the use of ultrafast diodes of the UF 4006-4007 series in the circuit. Conventional N4007 diodes do not work in this circuit. A capacitor standing in parallel with the transmitting coil needs a high-voltage (film) one. The throttle, standing in the power supply circuit, is used standard, from a computer power supply. A simpler solution, from the technical side, is the use of a blocking generator circuit. Such a device in its simplest form consists of only three parts – a transistor, a permanent resistor and a transmitting coil. As a key element, we used an IRF 630A transistor, the offset resistance can be selected in the range from 100 to 300 ohms. The transmitting coil is made with a midpoint and consists of 10 turns. The receiving coil is wound with a thinner wire and contains 25 turns. The appendix (Sheet V, Fig. 9-10) shows a schematic diagram of the demonstration and charger, and photos of the manufactured version. The disadvantages of the previous schemes are relatively unstable frequency characteristics, since neither a multivibrator nor a blocking generator can provide a stable waveform. In this regard, it was decided to make an experimental version of the generator with a master circuit. As a master frequency generator, the simplest option is to use the widely used NE 555 timer chip. It is switched on according to the standard generator circuit. The frequency-setting circuit is two resistors with a nominal value of 1 Kohm and a capacitor. The master generator controls a powerful key transistor included in the transmission coil circuit. The receiving unit is assembled according to a standard scheme using a linear stabilizer 7805. A diagram of the device and a photo of the experimental unit are given in the appendix (Sheet V, Fig. 11). The functional capabilities of the manufactured devices were analyzed, and conclusions were drawn based on the analysis, and graphs of the dependence of voltage and current on the distance between the coils were constructed. The voltage data on the receiving coil without load and the stabilization circuit, depending on the distance to the transmitting circuit, are shown in the graph in the appendix (Sheet VI, Fig. 12). In the load mode (the standard voltage of consumers is 12 V), the operability of the devices was tested on two incandescent lamps – a conventional 5 W and a halogen 24 W. As a result of the experiments, graphs of the dependence of the current consumed on the distance between the receiving and transmitting coils were constructed. Based on these graphs, it is very easy to calculate the actual voltage coming to the consumer when the coils interact:
P = UI, U = P/I
Graphs of the studied interaction processes and measured values are presented in the appendix (Sheet VI, Fig. 13-14). The next stage of research was the actual charging mode of a standard mobile device battery. During these studies, a circuit for stabilizing the output voltage on the chip of the linear stabilizer 7805 was used. During these studies, it turned out that at close distances between the receiving and transmitting coils, the current strength during charging is quite large (up to 4.5 A), which leads to the failure of the chip. As a result of the research, the optimal distance between the transmitting coil and the consumer was determined in the range from 2 to 4.5 Cm, which corresponds to a charging current from 2 to 1 A. At large distances, charging is impractical, since there is a sharp drop in the current mode. The identified parameters are shown in the graph of the application (Sheet VI, Fig. 15). In general, all circuits are functional, but less stable indicators of the circuit assembled using a blocking generator.
Ways to increase the distance of wireless power transmission
As can be seen from the above material, the distances of power transmission without wires are very small (do not exceed 4-5 cm). We have set ourselves the task of achieving, with wireless power transmission, large distances between the receiving and transmitting coils. In this case, it seems to us (based on the literature and Internet sources studied), based on the principles of wireless energy transmission, there are several ways to go:
Increasing the power of the transmitting generator.
Experimental selection of frequency characteristics of the generator.
Tuning the receiving and transmitting circuits into resonance.
Experiments with the shape and size of the transmitting and receiving coils.
All these paths can be used together and optimize the device parameters.
Initial experiments to increase the distance of wireless transmission of electricity
Initially, we were faced with the task of creating a powerful pulse generator for the operation of the transmitting oscillatory circuit. As a basis for the circuitry of a powerful generator, we decided to take a variant of a multivibrator. The choice is justified by the simplicity of the scheme and the easy adjustment of frequency characteristics. Along with the disadvantages (not quite stable operation in frequency), there is an undeniable advantage in tuning. As power switches, powerful IGBT transistors were used, designed for a voltage of at least 800V and currents up to 80A (naturally in pulse mode). The scheme of the device is generally accepted and has no fundamental innovations, except for the selection of time-lapse circuits (Appendix Sheet VII, Fig. 16). To control the power supply, an LED indicator was used in the circuit. The frequency characteristics of the generator are in the range of 0.15 – 0.2 MHz. Resonance tuning was carried out by selecting capacitors parallel to the coil, frequency characteristics by selecting resistances in the gate circuits of IGBT transistors. The supply voltage of the generator is 24V, from a 600W switching power supply. For experiments, we have chosen the MI (magnetic-inductive) technology as easier to manufacture. The shape of the coils is flat, a thin (3mm) copper tube was used for their manufacture. The winding of the coils is spiral. An inductor is a passive component of electronic circuits, the main purpose of which is to conserve energy in the form of a magnetic field. The property of an inductor is somewhat similar to a capacitor, which stores energy in the form of an electric field. Inductance (measured in Henry) is the effect of the occurrence of a magnetic field around a conductor with a current. The current flowing through the inductor creates a magnetic field that has a connection with the electromotive force (EMF) that counteracts the applied voltage. The resulting counteracting force (EMF) resists the change in alternating voltage and current in the inductor. This property of an inductive coil is called inductive resistance. It should be noted that the inductive resistance is in the opposite phase to the capacitive reactance of the capacitor in the AC circuit. By increasing the number of turns, the inductance of the coil itself can be increased. The energy stored in the inductor is equal to the expended energy required to ensure the flow of current I in the counteraction of EMF. The amount of stored energy in the inductance can be calculated using the following formula:
where L is the inductance, I is the current flowing through the inductor. The inductance of a flat coil is calculated by the formula:
where L = inductance in MCN, r = average radius of the coil, N = number of turns, d = depth of the coil. The inductor has a series resistance created by the copper winding of the coil itself. This series resistance converts the electric current flowing through the coil into heat, which leads to a loss of induction quality, that is, Q-factor. Q-factor is the ratio of inductance to resistance. The Q-factor of the inductor can be found through the following formula:
where R is the intrinsic resistance of the winding.
x=(-b±√(b^2-4ac))/2a
The inductance practically does not depend on the diameter of the wire. But in high-frequency coils, they strive to obtain the minimum resistance of the conductor in order to increase the Q factor (the ratio of inductive resistance to active). To this end, it is necessary to increase the diameter of the wire, but only for flat coils, since increasing the length of the winding (vertical) reduces the inductance. With a close, multi-layered arrangement of the coils, the effect of "displacement" of the current from the winding is observed, which increases the resistance. This effect is similar to the displacement of current, at high frequencies, in any conductors. As a result, the current flows only in a thin skin layer, at the surface of the conductor. The thickness of the skin layer decreases, and the resistance of the wire increases proportionally to the square root of the frequency. To obtain maximum Q-factor and inductance, it is more profitable to make the coil short (winding depth), but of a large diameter, with a D /l ratio of about 2.5. The inductance of such coils is more accurately calculated by an empirical (empirically selected) formula:
L = 0.1D2N2/ (4D + 11 l)
where the dimensions are taken in centimeters, and the inductance is obtained in microgenres. The same formula is applicable for a spiral or basket flat coil. The transmission coil in our design has a diameter of 400 mm., the number of turns is 7, the wire diameter is 3 mm. Calculations show that the inductance of this coil is 48 MCG. The energy stored in the coil is (at I = 20-25A, based on the power consumption) approximately 0.0096 J. The resonance frequency of the transmitting oscillatory circuit is determined by the formula:
f=1/((2π√LC)))
After making calculations, we obtain a resonance frequency of 0.0283 MHz (taking into account the capacitance of the capacitor 660000rF, and the inductance of the coil 48 MCG). The calculation table and the design of the receiving coil are given in the appendix (Sheet VII, Fig. 17-18). Based on these parameters, we determine the size and parameters of the receiving coil. The receiving coil has a diameter of 250 mm., the number of turns is 21. After making calculations, we determine the inductance at 266.8 MCG. We determine the capacitance of the capacitor of the oscillatory circuit of the receiving coil – 118544rF (0.12 UF) (Appendix Sheet VII, Fig. 19). Calculations are carried out on a calculator, on the Internet. During the experimental verification, we determined the distances of effective energy transfer and carried out measurements of the quality indicators of the received energy. Graphs based on the measurements of the parameters are given in the appendix (Sheet VIII, Fig. 20-23). The efficiency coefficient was calculated relative to the parameters of the current and voltage on the transmitting coil to the parameters of the current and voltage (under load) of the receiving coil.
Experiments with receiving and transmitting coils
We were prompted to further experiments on changing the parametameters of the receiving and transmitting coils by experimental work with single-turn vibrators (Appendix Sheet IX, Fig. 24). Using such devices, we have achieved, with their matching in resonance, approximately the same energy transfer distances as with multi-turn systems. The idea of creating multi-turn coils with dense winding, like a ring vibrator, arose. Calculations have shown that the inductance of such coils (tight winding and smaller diameter) increases sharply. In our case, the transmitting coil having an average diameter of 176.2 mm and 7 turns has an inductance of 229.4 µgn. The receiving coil, which has an average diameter of 149.5 mm, and the number of turns is three times larger – 21, has an inductance of 1261.7 ΜGN. In accordance with the above calculations, we determine the frequency of the resonant system (based on the capacity of the frequency–setting capacitor 0.8 UF) - 0.0117 MHz. Based on these data, we determine the capacitance of the capacitor of the resonant system of the receiving coil – 0.14 UF (Appendix Sheet IX, Fig. 25). Preliminary calculations of the entire system showed an increase in the power transmission range by at least 3-4 times compared to the previous version of the transceiver. Experimental tests of the system have yielded real results, stable energy transmission at a distance of up to 2 -2.5 meters. A photo of a working copy of a wireless power transmission system is given in the appendix (Sheet IX, Fig. 26), the main characteristics (Appendix sheet X, Fig. 27-30).
Conclusions
As a result of the work done , the following conclusions can be drawn:
The available literature and Internet sources on the issues of wireless energy transmission by induction method have been studied.
The principles of operation of high-power inverters are studied and circuit solutions for their construction are considered.
The analysis of the previous experience of manufacturing wireless power transmission systems without wires is carried out.
An experimental transceiver device of high power and acceptable frequency characteristics has been manufactured for conducting experimental studies. The parameters of the transceiver coils (antennas) have been determined to increase the range of wireless energy transmission.
Experimental studies have been carried out to determine the parameters of wireless energy transmission.
It is also worth noting that the further development of the project topic will be able to co-ordinate the energy sector in the future: electric vehicles will begin to displace cars using fossil fuels (thanks to the simplification of their charging: special roads will charge transport while it moves along them), which means the amount of exhaust gases in the atmosphere will decrease; power lines can be replaced with structures, capable of transmitting electricity wirelessly for many kilometers (it will be possible to realize the idea of Nikola Tesla); there will be new design solutions in the field of illumination: we will get closer to the ideas of the houses of the future, which were described in the past by science fiction writers; and in general, life without a heavy pile of various wires will become a little easier.
References and Internet sources
1. D. V. Sivukhin, General course of physics. — Ed. 4th, stereotypical. — M.: Fizmatlit; MIPT Publishing House, 2004
2.http://www.naexamen.ru/otvet/11/fizika/884.shtml, Laws of electromagnetic induction.
3. Tamm. Fundamentals of the Theory of Electricity, 9th ed., M., 1976.
4.M. Faraday, Experimental studies on electricity. Volume 1. Translated from English by E.A. Chernysheva and Ya.R. Schmidt-Chernysheva. Publishing House of the USSR Academy of Sciences, 1947.
5.http://www.scorcher.ru/art/theory/cosinov/tesla.htm , Wireless power transmission.
6.L. Barash, Wireless power transmission, Science and Technology, Science, http://www.membrana.ru/particle / 2007.
7.http://altinfoyg.ru/index.php/energetika/analiz/ipe.html , Induction energy transfer.
8.http://hiendflow.do.am/publ/obzory_tekhnologij/indukcionnaja_peredacha_ehnergii/2-1-0-1, Induction energy transfer.
9.http://electrik.info/main/fakty/918-sposoby-besprovodnoy-peredachi-elektroenergii.html - Methods of wireless power transmission.
10.http://www.russianelectronics.ru/leader-r/review/doc/70732/ - Wireless charging technology: operating principle, standards
11.https://masterok.livejournal.com/3054002.html - How wireless charging works.
12.http://www.russianelectronics.ru/leader-r/review/doc/70732/ - Principles of wireless power transmission. Standards.
13.https://bourabai.ru/toe/coils.htm - Properties of inductors.
14.http://twt.mpei.ac.ru/MCS/Worksheets/ET/p-149-1.xmcd is the inductance of flat coils.
15.http://coil32.ru/calc/flat-spiral-coil.html - Calculation of the inductance of a flat spiral coil.
16.http://tel-spb.ru/lc.html - Calculator for calculating the resonant circuit.
17.https://habrahabr.ru/post/219857/ - Wireless transmission of energy over long distances.